Little by little, wean yourself. This is the gist of what I have to say. From an embryo whose nourishment comes in the blood, move to an infant drinking milk, to a child on solid food, to a searcher after wisdom, to a hunter of invisible game.
Rumi
September 29th: We drove 45 minutes down to the Paul Scherrer Institut in Villigen Switzerland. I picked up my previously-requested badge and dosimeter, and got a tour of where I might be performing my experiment next year.


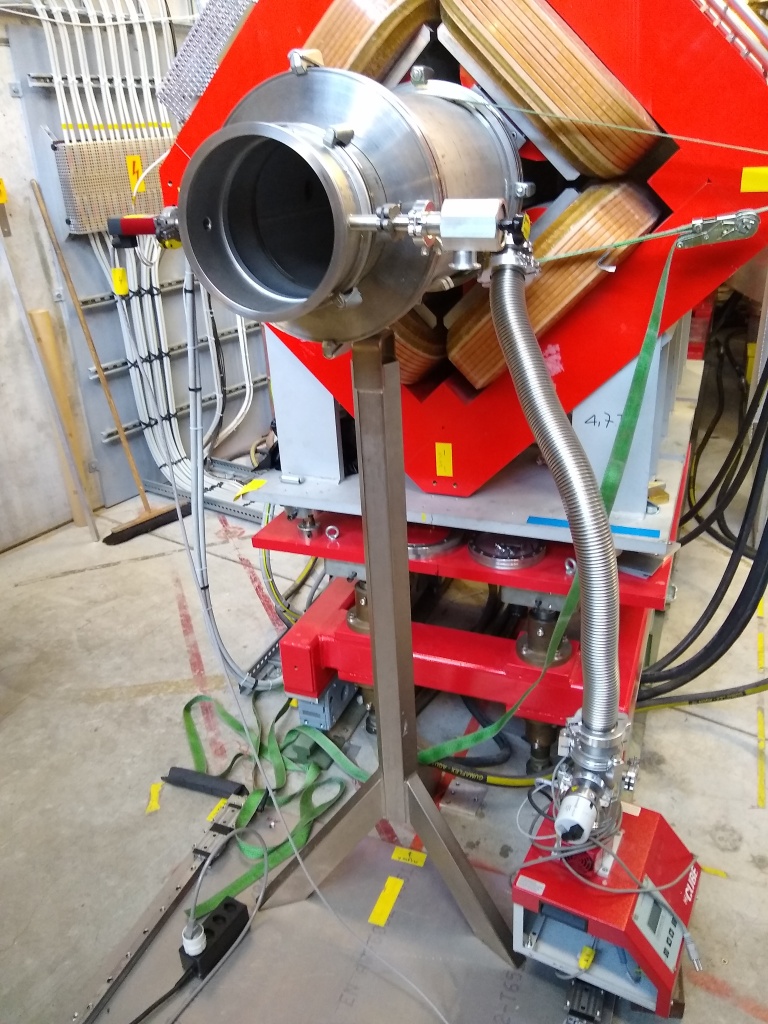


The particles were first called mesotrons (1936), then yukons after the particle predicted by Hideki Yukawa, then mu mesons after pi mesons were discovered, then muons after it was realized that they weren’t mesons at all but rather leptons, and that the real yukons were the pi mesons, which are mesons but still got shortened to pions. They were the first subatomic particles discovered that were not a component of atoms and thus seemed totally unnecessary, leading Isadore Rabi to quip “Who ordered that?”.
Anyway, I learned a lot about what I need to get done in the next couple of months before submitting the experimental proposal by January 10th. On the positive side, both Aldo (who gave me the tour) and Frank (who joined us for lunch) seemed positive about the experiment. Maybe they are getting a glimpse of how exciting it would be if this were correct.
On the negative side, there are lots of new issues to deal with, like getting insurance coverage, finding a second person to work with me, figuring out how to ship everything to Europe (and deal with customs etc.), running on Swiss 220V, dealing with beam impurities (including at least 10% electrons), and converting everything from wifi to hardwired ethernet (including optical ethernet links to get out of the sphere).
My biggest asset is that I am totally committed to making this happen. Whatever it takes, I will do.
Until one is committed, there is hesitancy, the chance to draw back, always ineffectiveness. Concerning all acts of initiative (and creation), there is one elementary truth, the ignorance of which kills countless ideas and splendid plans: that the moment one definitely commits oneself, then Providence moves too. All sorts of things occur to help one that would never otherwise have occurred. A whole stream of events issues from the decision, raising in one’s favour all manner of unforeseen incidents and meetings and material assistance, which no man could have dreamt would have come his way.
William H. Murray
But of course, we eventually had to leave for Bern. Our hostel was near the Thai embassy, so, after checking in, we had dinner at a Thai restaurant. Our theory was that the embassy wouldn’t allow it to be mediocre.
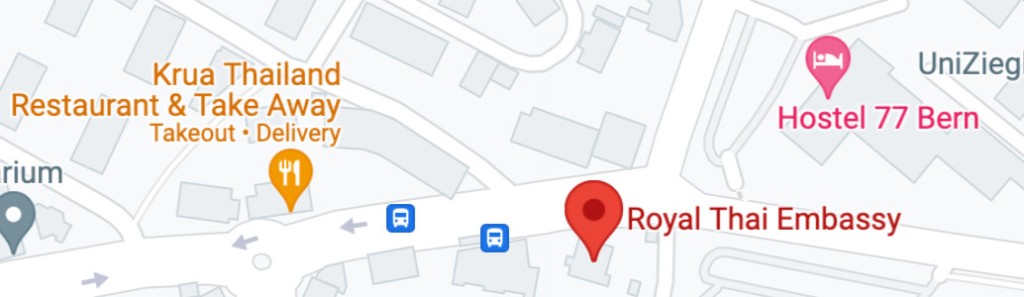

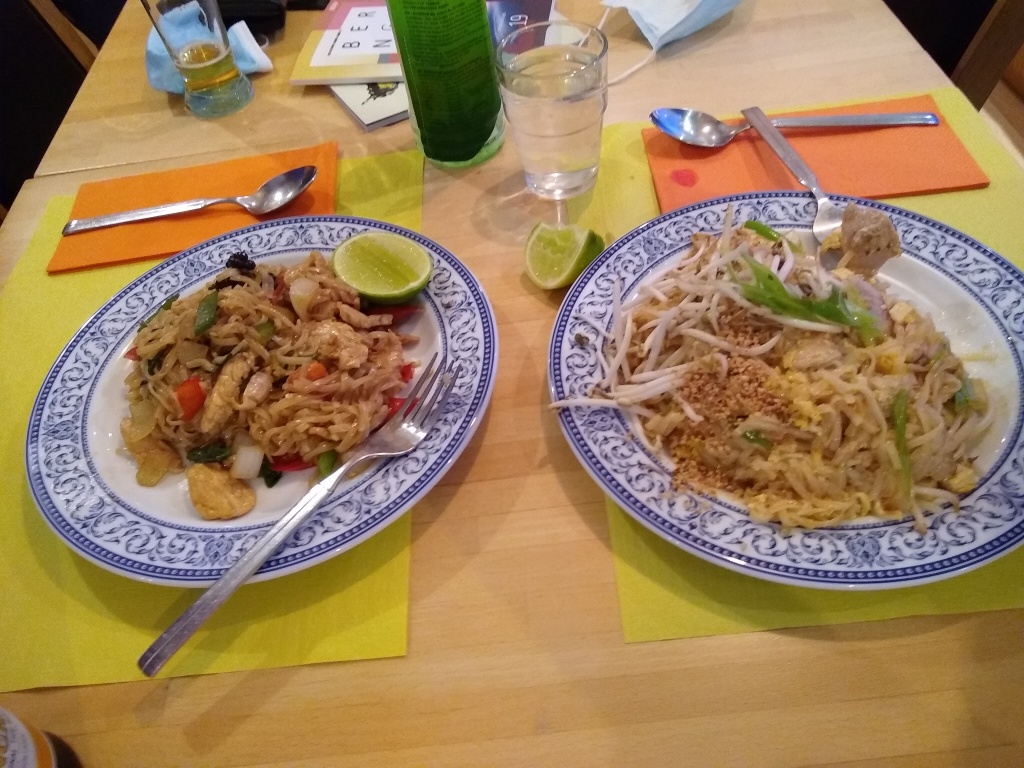
Hostel 77 was easily the most expensive lodging of our trip, but was quite basic (e.g. shared bathrooms). It did come with a decent free breakfast though. Still, Switzerland is far too pricey to be a good place to retire on a fixed income.