I submitted version 5.1 of “The magnitude of electromagnetic time dilation” paper to General Relativity and Gravitation on Isaac Newton’s birthday. You can download it from ResearchGate here; I haven’t updated Vixra yet.
Since I can’t do much on the theory side until GeRG gets back to me, I’ve been thinking about the experiment more. In particular, the size of the plastic scintillator needed.
There are two main criteria. First, we need to be able to stop muons in the scintillator, and detect when they arrived. And second, we need to detect when they decay.
The thickness needed for stopping is pretty easy to simulate crudely using SRIM/TRIM software on Windows. For example, let’s assume we’re trying to use the $139 kit from iradinc on Ebay:
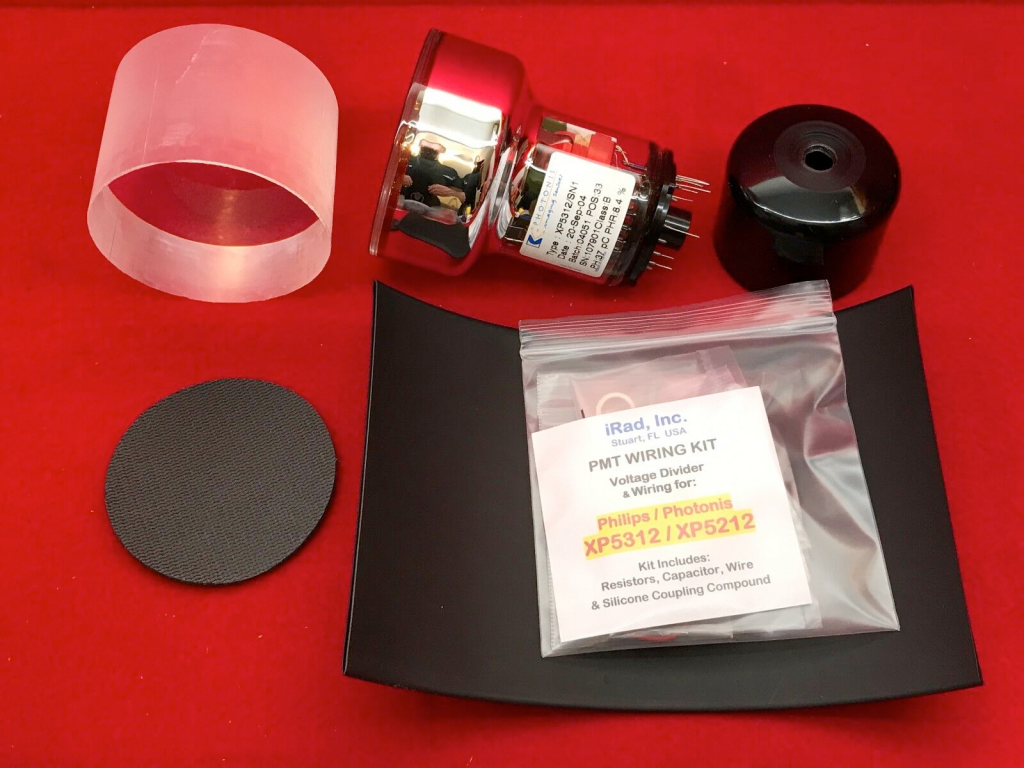
which has a 3″ (76 mm) diameter by 2.25″ (57 mm) thick chunk of BC-412. If we assume a 29 MeV beam in a vacuum, maybe in a non-conductive PVC pipe, it’ll first have to get out of the pipe and into the air that the rest of the experiment lives in. So we get a layer list that looks something like:
- 5.0 mm PVC (end cap of vacuum tube)
- 2.5 mm air (gap to VDGG sphere)
- 1.5 mm stainless steel (the sphere)
- 26 mm air (gap inside sphere to scintillator)
- 57 mm polystyrene (scintillator is actually polyvinyltoluene, but that’s not in the SRIM material list, so we substitute a different plastic with similar elemental composition and bond structure and density)
Simulating this in SRIM, using “H ions” (protons) with mass adjusted to 0.1135 amu to represent antimuons, and running 1,000 simulations, we get:
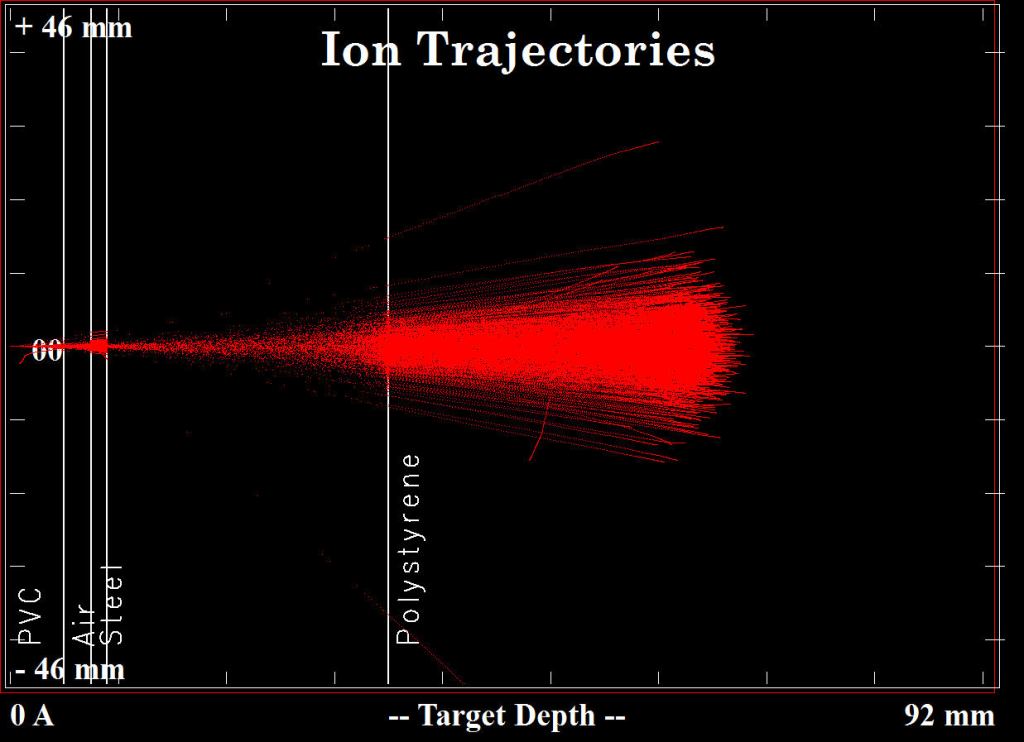
The average implantation depth is 64.0 +- 1.94 mm, which (since the plastic starts at 35 mm) means 29 mm into the 57 mm of scintillator, which is almost dead center (57/2 = 28.5 mm). So the beam energy is almost perfect. And a total scattered beam diameter of roughly (5.10 + 4.44)*2 = 19 mm means almost all of the muons will hit the 76 mm diameter scintillator. We’d probably lose about 1 in 1000 muons to a hard recoil that prevents it from reaching the scintillator (you can see one such recoil in the PVC above), and maybe 2 in 1000 to medium recoils that deflect it enough so that it passes in through the front edge of the scintillator but then out the side. Zero muons come anywhere close to exiting the back side of the scintillator; they just don’t have enough energy to penetrate all of it. So about 99.7% of the beam muons will stop in the scintillator, and they will dump most of their energy into producing photons.
So far so good. We can even reduce the radial scatter by cutting a hole in the sphere, so that the beam never hits the steel. (That would increase the implantation depth too.)
But a problem arises when considering the decay pulse. A muon (antimuon) decays into an electron (positron) and two neutrinos:
𝜇⁻ ⟹ e⁻ + 𝜈𝜇 + 𝜈̅e
𝜇⁺ ⟹ e⁺ + 𝜈̅𝜇 + 𝜈e
The neutrinos escape, taking all their energy with them. The electron/positron gets a random kinetic energy of up to about half the muon’s mass-energy of 105.7 Mev, following the Michel spectrum. So, maximum about 53 MeV and average about 40-45 MeV.
So at first glance, it seems like we should want a scintillator big enough to stop every electron and convert the maximum possible energy into photons. In the best possible case we can assume that the decay happens in the exact center. Thus the diameter needed would be twice the “depth” of a 53 MeV electron.
However, when I calculate that depth, the CSDA range for a 53 MeV electron is about rCSDA = 22.008 g/cm2, and the density of PVT is about dPVT = 1.05 g/cm3, so we get an expected range of r = rCSDA/dPVT = 22.008 g/cm2 / 1.05 g/cm3 = 20.96 cm. That means we would need a scintillator at least 42 cm diameter and 42 cm deep. That’s about (𝜋 × 21cm × 21cm × 42cm) / (𝜋 × 3.8cm × 3.8cm × 5.7cm) = 225 times as much plastic as in the iradinc kit. That would be expensive, costing perhaps $5-10K.
But something’s off here. The Teachspin student muon lifetime device is smaller than that, and I know it works because I’ve used it. The whole scintillator plus PMT plus HV supply fits in a cylinder that’s only 16.5 cm diameter x 36 cm tall:
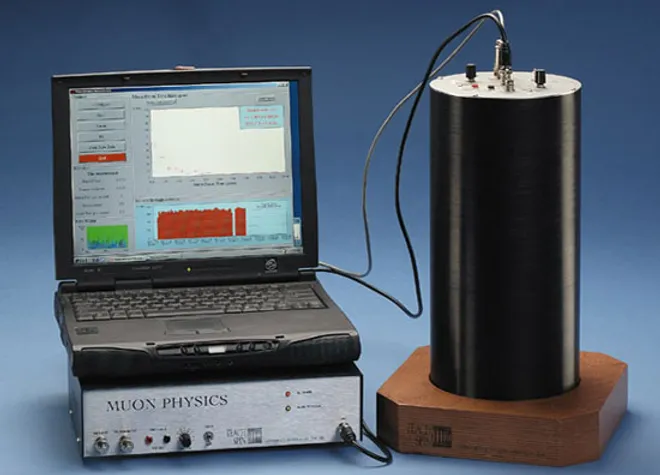
so the scintillator inside that is probably only about 16 cm diameter x 18 cm tall. That’s only 1/16 the volume calculated above.
Let’s take a step back. We want to detect both the muon stopping pulse and the muon decay pulse. Since both hit the same PMT, and we will use a level discriminator to reject noise, it would be most convenient if both pulses were approximately the same height. Crudely, that means that both pulses deposit about the same amount of energy into the plastic.
So if the beam energy is 29 MeV, we don’t need to capture all (up to) 53 MeV of the decay pulse. We only need to capture the first 29 MeV of it. If the rest escapes, that’s fine.
So if the TOTAL range at 53 MeV is 21 cm, we need to find the range of a (53 – 29) = 24 MeV electron, and subtract that off. From the same tables as above (https://physics.nist.gov/cgi-bin/Star/e_table.pl) we get about 11.4 g/cm2 and thus a range of 11.4 g/cm2 / 1.05 g.cm3 = 10.9 cm. So that means we only need the first 21.0 – 10.9 = 10.1 cm, giving a roughly 20 cm diameter x 20 cm thick scintillator. This is a little larger than the Teachspin one, so we can be certain it’s adequate.
I guess that gives me some rough parameters. Maybe it’s time for g4beamline simulation.